ESG Economist - Can European natural gas infrastructure be used for CO2?
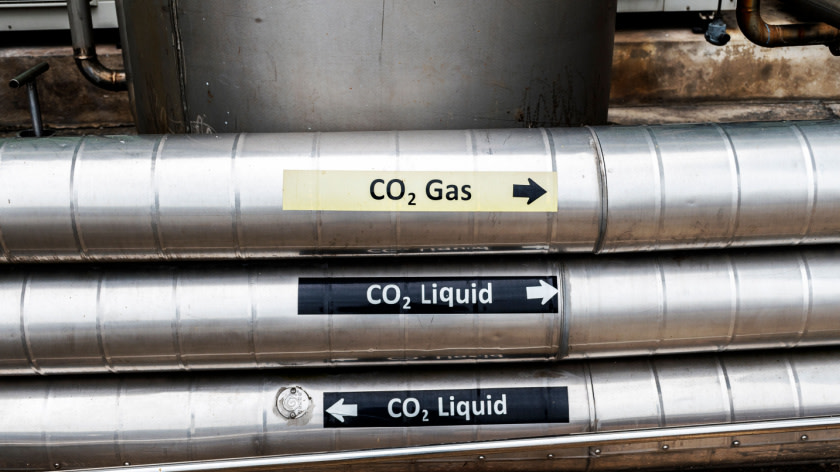
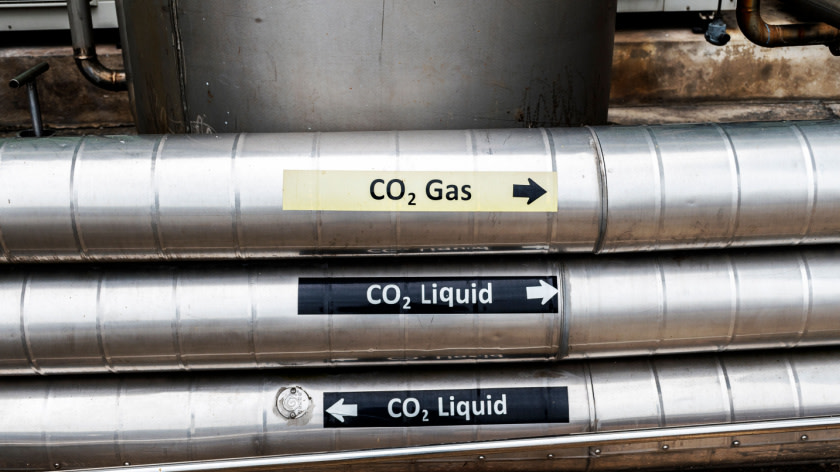
The natural gas infrastructure needs to be adjusted to deal with possible corrosion, CO2 impurities and differences in pressure and may not be located in the right place for transporting CO2. Therefore, there is the need for new infrastructure to be capable of accommodating EU-wide requirements associated with CO2 transportation. There are around 100-120 potential CO2 capture clusters and about 100 storage sites throughout Europe. The future European CO2 transport network could reach a length of 6,700-7,300 km by 2030, and might extend to between 15,000 and 19,000 km by 2050. Its deployment could cost between about EUR 6.5 billion and EUR 19.5 billion by 2030, rising to between EUR 9.3 billion and EUR 23.1 billion in 2050.
Carbon capture and direct air capture have an important role to play in our quest to net-zero, to compensate for the residual CO2 emissions from the hard to abate sectors
In Fit-for-55 the aim for carbon capture was 50 Mtpa by 2030, 114 Mtpa in 2040 and 245Mtpa in 2050
In the new proposals from the EC for 2030 and 2040 these goals are more ambitious, namely 58.8 Mt per annum by 2030 and 243 Mtpa in 2040 in a 90-95% reduction scenario
But there needs to be an infrastructure to transport and store (if not utilized) this CO2
A key question is whether the existing natural gas infrastructure can be used for CO2
The natural gas infrastructure needs to be adjusted to deal with possible corrosion, CO2 impurities and differences in pressure and may not be located in the right place for transporting CO2
Therefore, there is the need for new infrastructure to be capable of accommodating EU-wide requirements associated with CO2 transportation
There are around 100-120 potential CO2 capture clusters and about 100 storage sites throughout Europe
The future European CO2 transport network could reach a length of 6,700-7,300 km by 2030, and might extend to between 15,000 and 19,000 km by 2050
Its deployment could cost between about EUR 6.5 billion and EUR 19.5 billion by 2030, rising to between EUR 9.3 billion and EUR 23.1 billion in 2050
Introduction
Last year we did a deep dive into carbon capture (). We focussed on technologies that capture emissions from combustion. Those are the CC (carbon capture) and CCUS (carbon capture, utilisation and storage). In June we published a report on geo-engineering carbon sequestration technologies where we discussed technical (direct air capture), geological (enhanced weathering/mineralization) and biological carbon sequestration. Carbon capture and direct air capture have an important role to play in our quest to net-zero, to compensate for the residual CO2 emissions from the hard to abate sectors. According to the IEA, the global CO2 captured in 2022 was only 0.04 Gt. The graph below shows the IEA's STEPS, APS and NZE scenarios. STEPS is the stated policies scenario, APS is the announced policies scenario and NZE the net zero scenario. In a net zero scenario (NZE), global CO2 captured would need to be around 1 Gt CO2 in 2030 and around 6 Gt CO2 by 2050 (see graph below). This is still negligible compared to the global CO2 emissions of 37.4 Gt CO2 in 2023. But there needs to be an infrastructure to transport and possibly store (if not utilized) this CO2.
Can the existing natural gas infrastructure in Europe be used for CO2 transport and storage? This is the main question we tackle this report. Before we can answer this question, we first delve into the specific characteristics of CO2 and how to transport and store CO2. What are the challenges of transporting and storing CO2. What are the EU plans and goals for CO2 transport and storage? We end with a conclusion.
CO2 characteristics
For transporting CO2 the state of CO2 is very important. CO2 usually behaves as a gas in air at standard temperature and pressure or as a solid called dry ice when frozen. CO2 in the gas state is transported at close to atmospheric pressure and occupies such a large volume that very large facilities are needed (see more ). CO2 occupies less volume if it is compressed, and compressed CO2 is transported by pipeline. CO2 takes up less volume when it’s compressed and even less when it is liquified, solidified or hydrated. Therefore, CO2 is often compressed and liquefied to supercritical state. Supercritical CO2 is a state of CO2 where distinct liquid and gas phases do not exist. This is done at a pressure greater than 74 bar and a temperature higher than 31°C (304 Kelvin, see graph below).
There is also the possibility to transport CO2 in a liquid state with the right temperature and pressure conditions. This solution requires a good isolation of the pipes.
Ways to transport CO2
CO2 can be transported via pipelines, ships, road and rail. The transportation of CO2 via pipelines is a mature technology. It is most common and is less costly. The CO2 is preferably dry and free of hydrogen sulphide because at this state, the corrosion of pipelines is then minimal. Pipeline transportation of CO2 over longer distances is most efficient and economical when the CO2 is in the dense phase. CO2 also can be transported as a liquid in ships, road or rail tankers that carry CO2 in insulated tanks at a temperature well below ambient, and at much lower pressures. The average-sized LPG tanker could carry approximately 45,000 tonnes of CO2. Shipping is a mature technology for liquefied natural gas (LNG) and liquefied petroleum gas (LPG) but is not widely used for CO2 transportation today. LPG tankers are a closer analog for CO2 transport via ship than LNG tankers because liquefied CO2 must be transported at elevated pressures like LPG, whereas LNG is transported at atmospheric pressure. LPG tankers can be repurposed for CO2 or dual-purpose transport, but in general, tankers specifically designed for CO2 transport can be better optimized for maximum capacity and investment cost ().
Challenges to transport CO2
Corrosion
Dry carbon dioxide does not corrode the carbon-manganese steels, that is generally used for pipelines, as long as the relative humidity is less than 60%. Moisture-laden CO2, on the other hand, is highly corrosive, so a CO2 pipeline in this case would have to be made from a corrosion-resistant alloy, or be internally clad with an alloy or a continuous polymer coating. Some pipelines are made from corrosion-resistant alloys, although the cost of materials is several times larger than carbon-manganese steels. So the pipes (and the tanks) must be kept free from corrosion. This problem can be easily solved by purification, a thorough dehydration and the use of corrosion suppressant. Dehydrating the CO2 entails removing the water from the gas mixture stream ).
Impurity
The captured CO2 is never 100% pure. Purity depends on the nature of the fuel (oil, natural gas or coal) and the capturing technique (postcombustion, oxycombustion or precombustion). Different heavy metals can be found in the gas mix with CO2. The presence of certain impurities in CO2, such as methane and nitrogen, can lead to reduced pipeline capacity. Indeed, higher methane levels require greater pumping/compression. The presence of impurities shifts the boundary towards higher operating pressures to keep the CO2 in the supercritical or dense phase. Furthermore, the impurities can lower the density of CO2, which also lowers the storage capacity for the CO2.
Pressure
The feasibility of repurposing natural gas pipelines for CO2 transport is not practical for transporting large quantities of CO2 over long distances. This is because CO2 requires a higher pressure than natural gas to be kept in a liquid state for pipeline transport, and thus thicker pipelines are generally needed.
Use of existing natural gas pipelines
Can natural gas infrastructure can be used to transport CO2? Natural gas infrastructure needs to be adjusted to deal with possible corrosion, CO2 impurities and difference in pressure. So there is a possibility that the pipelines used for CO2 transport would need to operate under different conditions compared to most existing pipelines. Another important question to ask if the existing natural gas infrastructure is located in the right place for transporting CO2. As discussed below, the cost of CO2 storage is very site dependent and therefore suitable locations may not align with existing pipelines. Therefore, there is the need for new infrastructure to be capable of accommodating EU-wide requirements associated with CO2 transportation (more on this below).
Storing CO2
Carbon storage refers to the storage of the captured carbon in a deposit. Carbon storage is also called sequestration. There are two forms of sequestration namely biologic carbon sequestration and geologic carbon sequestration. Biologic sequestration refers to storage of atmospheric carbon in vegetation, soils, woody products and aquatic environments. Geologic carbon sequestration is the process of storing CO2 in underground geologic formations such as geological sinks, oil & gas reservoirs, saline formations, coals seams shales and basalt (). In this report, we focus on geological carbon sequestration. The CO2 is securely sequestered in the deposits through four trapping mechanisms ( and ). These mechanism prevent upward migration and leakage of CO2 (see graph below).
The vast majority of CO2 storage potential worldwide is in onshore and offshore saline aquifers (USGS, 2013). The cost of CO2 storage is very site dependent because the geologic characteristics vary from site to site and injection, labor, drilling, capital, and other costs vary regionally. A handful of geologic parameters are primary determinants of whether a reservoir is favourable for CO2 storage: permeability, thickness, depth, porosity, and lateral continuity ().
EU Industrial Carbon Management Strategy
The adoption of the European Green Deal, the European Climate Law and the subsequent proposals to increase energy and climate targets for 2030 have made carbon management technologies an important part of the EU decarbonisation effort. On 6 February 2024, the EC released its Industrial Carbon Management Strategy. This strategy focuses on three technological pathways.
Capture of CO2 for storage
Capture of CO2 for utilisation
Removal of CO2 from the atmosphere
The CO2 transport infrastructure is crucial for these three pathways. CO2 transport infrastructure projects are within the scope of the Trans-European Networks for Energy. They can apply to become projects of common interest (PCIs, see ) and subsequently apply for support under the Connecting Europe Facility (CEF). Projects of Common Interest are key cross-border infrastructure projects that link the energy systems of EU countries. To become a PCI, the project has to be necessary for at least 1 of the energy infrastructure priority corridors and areas mentioned in the revised TEN-E Regulation, have a significant impact on energy markets and market integration in at least 2 EU countries, boost competition on energy markets and help the EU's energy security by diversifying sources, as well as contribute to the EU's climate and energy goals by integrating renewables.
Every 2 years, the Commission adopts an EU list of PCIs and since 2023 also of projects of mutual interest (PMIs). PMIs are projects promoted by the EU in cooperation with countries outside the EU. They contribute to achieving the EU’s energy and climate objectives, as well as to their own countries’ sustainability objectives (s). In November 2023, the Commission adopted a new PCI list based on the revised TEN-E Regulation (), which also includes PMIs between EU and non-EU countries. This list includes 14 CO2 network projects across Europe. In April 2024 the EC published the first PCI/PMI list in the Official Journal of the European Union ().
EU goals for CO2
The Net-Zero Industry Act has an annual EU objective for CO2 storage of 50 Mt per annum (Mtpa). Modelling results for the EU’s 2040 climate target Communication indicate that approximately 280 million tonnes would have to be captured by 2040 and around 450 million tonnes by 2050. By 2040, close to half of the CO2 that is captured annually would have to come from biogenic sources or directly from the atmosphere (see the graph below, ).
iogenic CO2 refers to carbon in wood, paper, grass trimmings, and other biofuels that was originally removed from the atmosphere by photosynthesis and, under natural conditions, would eventually cycle back to the atmosphere as CO2 due to degradation processes.
In this report, we focus on the amount of CO2 to be captured and needs to be stored. This is the dark blue part in the upper part of the graph below and around 250 Mt per annum. This number will also be mentioned in the two scenarios below.
For successful deployment of CCUS, it is necessary to develop infrastructure for transporting captured CO2 from its sources to suitable storage sites. On 6 February the Joint Research Centre (JRC) published a report on Shaping the future CO2 transport network for Europe (). According to this report, the development of a European CO2 pipeline infrastructure will be challenging during the early phases of CCS deployment, before 2030, and alternative forms of CO2 transport should also be explored. The EU lacks commercially proven CO2 storage capacity in the early phase of CCS deployment. Coordination efforts are needed to identify suitable CO2 storage locations.
This study of the JRC identified around 100-120 potential CO2 capture clusters and about 100 storage sites throughout Europe. The study shows that the future European CO2 transport network could reach a length of 6,700-7,300 km by 2030, and might extend to between 15,000 and 19,000 km by 2050. Its deployment could cost between about EUR 6.5 billion and EUR 19.5 billion by 2030, rising to between EUR 9.3 billion and EUR 23.1 billion in 2050.
In this report, we focus on the Fit-for-55 for EU and the EC’s proposal of 90% to 95% reduction by 2040. The results are in the graphs below. The graph on the left shows the projected CO2 captured in Megaton per annum (Mtpa) under the two scenarios. As the 90-95% reduction goal of 2040 is more ambitious, this scenario shows a more rapid increase in projected CO2 captured between 2030 and 2040. The graph on the right shows total CO2 amount that is expected to be stored under the two scenarios by 2050. The total CO2 stored under the EC plan of 90-95% reduction is considerably larger.
The distribution of CO2 storage sites and capacities across Europe is not evenly spread. As a result, it will be necessary to develop storage sites beyond the North Sea and construct an extensive pipeline infrastructure spanning several EU Member States and neighbouring countries.
For the Fit-for-55 scenario
In the Fit-for-55 scenario with the proposed Net-Zero Industry act (NZIA) target of 50 million tonnes of annual CO2 storage capacity by 2030 requires approximately EUR 3 billion investments in carbon storage facilities, depending on the locations and capacity of the geological storage sites. Furthermore, a Commission report estimates investment needs for the transport infrastructure of pipelines and ships associated with the NZIA target to range between EUR 6.2 and 9.2 billion by 2030. Finally, the capture costs from point sources are estimated to range from EUR 13/t and EUR 103/t of CO2 depending on the industry, capture technology and CO2 concentration ().
The CO2 transport network extends to 17 countries, the total network length amounts to 6,000 km and there are 16 cross-border connections. There are seven active storage nodes in six countries. In 2040, there is a significant development of the network in Spain, Sweden and central Europe. The transport network is then about 8,700 km long and CO2 is captured in 43 active source nodes in 17 countries and stored in 18 active storage nodes in 10 countries. In 2050, the transport network extends to 21 countries with 25 cross-border connections. The total length of the network is about 15,200 km. CO2 is being captured in 120 active source nodes in 21 countries. It is being stored in 38 active sink nodes in 15 countries. The large number of countries where CO2 is stored indicates a significant spatial distribution of CO2 storage. However, the North Sea region remains the most important storage area. The longest parts of the network are located in France, Italy, Germany and Spain. Investment costs are EUR 9.5 billion for this scenario and these results are based on medium infrastructure costs. The average flow of CO2 per pipeline is 11.4 Mt per annum ).
90-95% reduction 2040
In the 2040 scenario with 90-95% reduction by 2040 most of the storage projects are situated in the North sea region, but there are also capture projects active in Greece, Bulgaria, Croatia, Austria, Italy and southern France. Between 2030 and 2040 there is a sharp increase in the implementation of the CO2 capture technologies (21 countries with 111 active storage nodes) and the total CO2 capture capacity increases to about 243 Mtpa. The total built CO2 transport network increases to about 15,400 km, but the used part of the network amounts to 14,800 km. The CO2 transport network evolves throughout the EU. There is one large network connecting central and western Europe and the North Sea region. In 2050, the CO2 transport network extends throughout 21 EU countries. About 250 Mtpa of CO2 is being captured in 114 active capture nodes, transported via a network of about 15,000 km with 22 cross-border connections and stored in 36 active storage nodes. The total investment costs are 11.2 bn and the average flow of CO2 per pipeline is 12.4 Mt per annum (). The graph below shows the projected CO2 captured and storage capacity per annum of this scenario up to 2031. Only in 2030 the storage capacity is expected to exceed the annual captured CO2.
Conclusion
Carbon capture and direct air capture have an important role to play in the quest to net-zero, to compensate for the residual CO2 emissions from the hard to abate sectors. The EU has set goals to increase CO2 storage. In Fit-for-55 the aim was 50 Mt per annum by 2030, 114 Mt per annum in 2040 and 245 Mt per annum in 2050. In the new proposals from the European Commission for 2030 and 2040 these goals are more ambitions, namely 58.8 Mt per annum by 2030 and 243 Mt per annum in 2040 in the 90-95% reduction scenario. But there needs to be an infrastructure to transport and store (if not utilized) this CO2. The main question is if the natural gas infrastructure can be used to transport CO2. The natural gas infrastructure needs to be adjusted to deal with possible corrosion, CO2 impurities and difference in pressure. Moreover the existing natural gas infrastructure may not be located in the right place for transporting CO2 because CO2 capture and storage could be located away from the natural gas infrastructure. Therefore there is the need for new infrastructure to be capable of accommodating EU-wide requirements associated with CO2 transportation. There are around 100-120 potential CO2 capture clusters and about 100 storage sites throughout Europe. Moreover the future European CO2 transport network could reach a length of 6,700-7,300 km by 2030, and might extend to between 15,000 and 19,000 km by 2050. Its deployment could cost between about EUR 6.5 billion and EUR 19.5 billion by 2030, rising to between EUR 9.3 billion and EUR 23.1 billion in 2050.