ESG Economist - Could carbon sequestration technologies help to reach net-zero?
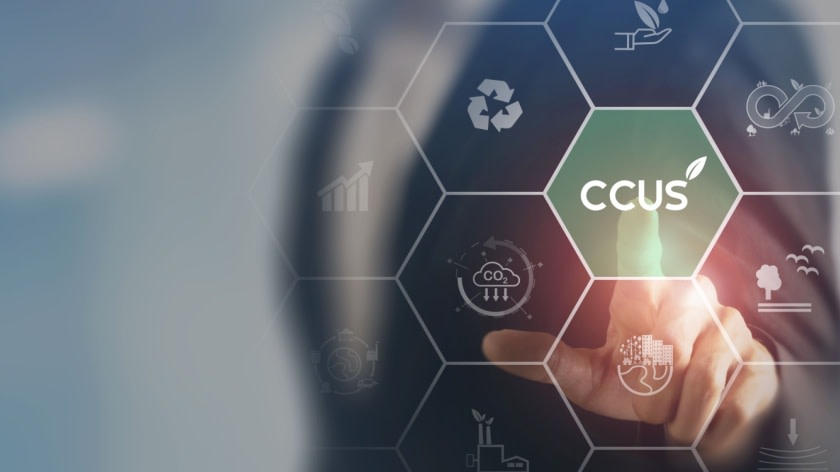
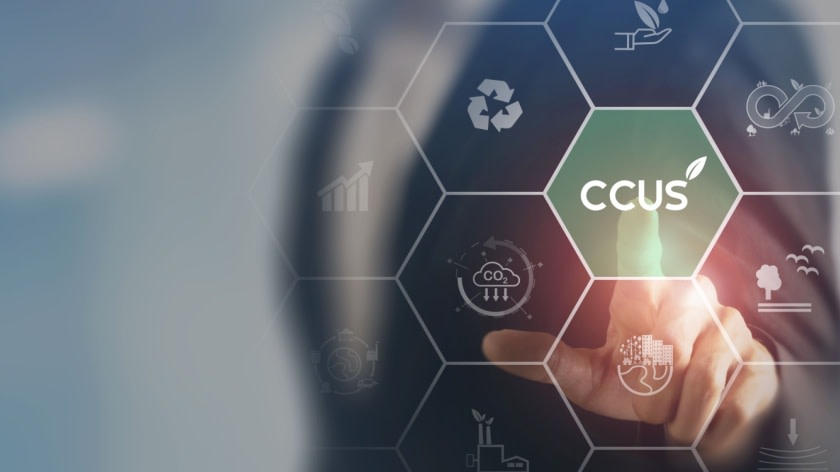
The goal is to reach net zero by 2050, to stay within the carbon budget for a pathway to stay below 2°C and pursue efforts to limit the temperature increase to 1.5°C above pre-industrial levels. There are several ways to achieve this goal. First, limiting and mitigating CO2 and other greenhouse gas emissions. Second, capture emissions from combustion (CCS). Third, capture CO2 and other emissions from the atmosphere if despite all the efforts there are still rest-emissions. Fourth other ways to manage radiation and to influence temperature rise. To reach the goal a combination of these ways is necessary. Removing CO2 from the atmosphere and radiation management fall into the category of geoengineering. In this report, we explain what geoengineering is. Then we focus on the carbon sequestration technologies and what they can do to help limit temperature rise. We end with a conclusion. In our next report we will focus on the other category of geoengineering, namely radiation management.
The goal is to reach net zero by 2050, to stay within the carbon budget for a pathway to stay below 2°C and pursue efforts to limit the temperature increase to 1.5°C above pre-industrial levels
There are several ways to achieve this goal. One way is to capture CO2 from the atmosphere and store it
There are several carbon sequestration technologies. Each technology has its pros and cons
Overall, most technologies are not ready to be implemented at a large scale and even if they are ready, they are still relatively expensive
The most attractive options in terms of costs are reforestation and afforestation
What is geoengineering
Geoengineering refers to a set of emerging technologies that could manipulate the environment and partially offset some of the climate impact change. Geoengineering is a term that covers a lot of technologies which generally fall under three categories: carbon sequestration technologies, solar radiation management and earth radiation management. In this report, we focus on carbon sequestration technologies. Carbon sequestration is the process of capturing and storing atmospheric carbon dioxide. This includes a wide spectrum of technologies that remove carbon dioxide from the atmosphere and store it afterwards. These technologies try to break the link from emissions to concentrations (see more ).
The carbon cycle
Before we move on to the carbon sequestration technologies, we first introduce the carbon cycle. The carbon cycle is the process that moves carbon between plants, animals, and microbes; minerals in the earth; and the atmosphere. Carbon in the form of carbon dioxide (CO2) is an important part of our atmosphere, where it helps to control the Earth’s temperature. Because only a tiny number of atoms reach the Earth from space, our planet is called a closed system. This means the Earth does not gain or lose carbon. But carbon does move constantly (see more ).
The temperature has increased because of excessive percentage of greenhouse gases (including carbon dioxide) in the atmosphere. To limit global warming, the increase of CO2 in the atmosphere also has to be limited (see more on carbon budget ). As the carbon cycle is a closed cycle, ideally, this carbon needs to be cycled away from the atmosphere towards plants, microbes and minerals in the earth. Carbon sequestration technologies have this goal. Removal is one thing and storage is another (see more ).
Carbon sequestration technologies
There are three types of carbon sequestration: biological carbon sequestration, geological carbon sequestration, technological carbon sequestration. Biological carbon sequestration is the storage of CO2 in vegetation such as grasslands or forests as well as in soil and oceans. Geological carbon sequestration is the process of storing CO2 in underground formations or rocks. Technical carbon sequestration are removal technologies that use CO2 as a resource. The table below provides an overview of the different technologies.
Technical carbon sequestration technologies
We start with Direct Air Capture. This is a technology that removes CO2 from the atmosphere for storage (see more ) or utilization (see more ). According to the IEA, twenty-seven direct air capture plants have been commissioned to date, capturing almost 0.01 Mt CO2 per year. If all the facilities were to advance (also the ones at concept stage) the deployment of direct air capture would nearly reach the level required in 2030 under the net zero emissions scenario by 2050 or around 62 Mt CO2/year. But this is still negligible compared to the global CO2 emissions of 37.4 Gt CO2 in 2023.
The graph sets out direct air capture CO2 removal pathways in three scenarios. The green line is Net Zero in 2050, the yellow line is the Announced Pledges Scenario or APS and the dark line Stated Policies Scenario or STEPS. In all these scenarios until 2030 the Direct Air Capture capacity is still insignificant compared to the total CO2 emissions globally. After 2030 the role of Direct Air Capture is increasing in absolute and relative terms because global emissions are declining in the Net Zero Scenario. In the other two scenarios the captured CO2 from the atmosphere via direct air capture remains less than 1% of the total annual CO2 emissions. So, capacity is limited.
Besides the fact that there is limited capacity, there is other disadvantage of direct air capture. It is more expensive compared to CCS as it is very energy intensive. This is mainly because of the low concentration of CO2 in the atmosphere compared to the concentration of CO2 in a flue gas for CCS. However, one major advantage is that the direct air capture installation can be placed everywhere, so also close to renewable energy capacity. The costs of this technology vary widely and ranges between 200 to 1,600 US dollars per ton CO2.
Geological carbon removal sequestration
Next to direct air capture there are also geological carbon sequestration technologies. These technologies are based on the natural process where certain minerals inside rocks react with atmospheric CO2 to create carbonates. Carbonates are solid minerals that securely remove and store CO2. This process is called weathering. Weathering can be accelerated. There are specific minerals that react with CO2 to make stable carbonates such as olivine or basalt. These minerals could be mined, grinded into powder and spread over the soil or dumped in the sea so that it can react with CO2. Another way is to expose powdered rocks to streams of pure CO2 from direct air capture facilities (see more ).
Some scientists estimate that up to per year could be removed from the atmosphere globally by 2035, and 10 gigatons of CO2 per year by 2050, if we invest sufficient funds in research and testing today to prepare for larger-scale deployment in the coming years (see more ). While other scientists estimate that this technology could be scaled up to capture 2–4 billion metric tons of CO2 (GtCO2) per year by 2050, with rates of more than 20 GtCO2 per year theoretically possible by 2100. So potentially this technology could sequestrate more CO2 than direct air capture and storage. But they could also be used together.
This technology has advantages and disadvantages. The advantages and disadvantages are often linked. To start the basic chemistry of enhanced mineralization is well understood, and the technology to mine, grind, and disperse rock is widely available. But research on enhanced mineralization as a form of carbon sequestration, however, remains in comparatively initial stages, with much more work to be done to evaluate its efficacy and social and environmental sustainability. Moreover, this technology could improve soil quality, but the other side of the coin is that the soil could be contaminated depending on the context and type of rock used. Furthermore, there are always social and environmental disadvantages to mining.
The cost estimates vary widely, from less than 50 US dollars per ton of CO2 sequestered to more than 200 US dollars per ton.
Biological carbon sequestration technologies
We discussed above direct air capture and enhanced mineralization. In this section we will focus on biological carbon sequestration technologies. These technologies use photosynthesis to trap carbon from the atmosphere. Then they process it for long term storage. Photosynthesis is the process by which plants use sunlight, water, and carbon dioxide to create oxygen and energy in the form of sugar. This sequestration can be done at a soil level, in the sea, by plants (and processing this) and by trees. Most of the “technologies” are already done by nature itself and we try to enhance and optimize these processes. We start with carbon sequestration by the soil.
Soil carbon pool sequestration
CO2 can be removed from the atmosphere and stored in the soil carbon pool. This process is called soil carbon sequestration. It is primarily mediated by plants through photosynthesis, with carbon stored in the form of soil organic carbon. The long-term conversion of grassland and forestland to cropland (and grazing lands) has resulted in historic losses of soil carbon worldwide, but there is a major potential for increasing soil carbon through restoration of degraded soils and widespread adoption of soil conservation practices. Improved agricultural practices can help mitigate climate change by reducing emissions from agriculture and other sources and by storing carbon in plant biomass and soils (see more ).
Ocean fertilization
Ocean fertilization is a theoretical sequestration technology that tries to improve ocean areas that currently have low productivity. It involves adding nutrients to the upper (sunlit) layers of the ocean to stimulate phytoplankton activity (photosynthesis) to draw down atmospheric CO2 levels. Phytoplankton need key elements like iron, nitrogen or phosphorus. So substantial amounts of micro-or macro-nutrients are dumped into these areas to stimulate the growth of phytoplankton. This plankton will then absorb atmospheric CO2 and store carbon. After blooming they die and sink to the ocean floor taking with them the CO2 they had photosynthesized into new tissues (see more ).
This technology has several disadvantages. First, some experiments have shown that the CO2 uptake by phytoplankton is much lower than predicted and that much of the carbon does not necessarily sink to the ocean floor (see more ). Second, this technology can reduce oxygen levels, changing the phytoplankton species (development of undesired algal blooms) and lowering biological diversity. Third, ocean fertilization can affect global nutrient distribution, where some areas can experience a reduction on nutrient supply (see more ). Last but not least, carbon produced through ocean fertilization will eventually go back to the atmosphere (see more ). So, this technology has a lot of negatives. Therefore, the UN convention on Prevention of Marine Pollution decided to strictly regulate ocean fertilization activities and de facto banning against all form of commercial deployment.
Artificial upwelling
Artificial upwelling is a theoretical carbon sequestration technology. The technology enhances the upward transport of nutrient-rich deep waters using pipes or wave pumps (artificial upwelling). This has a fertilizing effect and can make the waters of ocean deserts more productive. Through the stimulated growth of phytoplankton, more CO2 can be fixed in their biomass, which increases the carbon uptake of the upper ocean. When the phytoplankton die and their biomass sinks into the deep ocean, the fixed carbon can be stored at depth for 100 years and more (see more ). But various technologies have been tested without much success. This technology seems to have limited sequestration potential. It requires plastic pipes that reach hundreds of meters deep often in combination with platforms. The structures could if installed on larger scale cause serious issues for marine life, shipping and fisheries.
Afforestation and reforestation
Another way to sequester carbon is through afforestation and reforestation. Afforestation is converting long-time non-forested land into forest. So, this is the establishment of forests where previously there have been none, or where forests have been missing for a long time (50 years according to UNFCCC). Reforestation is the process of specifically planting native trees into a forest that has decreasing numbers of trees. The increased number of trees helps to reduce atmospheric carbon dioxide.
There are many advantages of afforestation and reforestation. More trees improve ground water quality, prevent flooding, reduce soil erosion and to create new wildlife habitats. But there are also some disadvantages. Planting trees must be applied on a very large scale to have a significant impact. Moreover, if unsustainably managed they may lead to the destruction of original non-forest eco-systems (see more ) and the land used can’t be used for other uses.
The costs for reforestation are relatively low and vary between 10 and 60 US dollars per ton captured CO2.
Biomass carbon removal and storage, enhanced photosynthesis
We have already mentioned several biological carbon sequestration techniques. The last one we focus on may play a key role in the future. It is the role of plants and what can be done with plants. Harvested plants hold a lot of carbon but it is not durable in that form. One way to store it for the long term is burial or sinking. The other route is to put biomass into a kind of pressure cooker at high heat. This can take that biomass and create durable solids such as biochar, liquids such as bio-oil and gases (carbon dioxide) for storage or utilization.
Biomass is heated in the absence of oxygen or under low oxygen conditions. This process is called pyrolysis. Because no oxygen is present combustion does not occur, rather the biomass thermally decomposes into combustible gases and biochar. Most of these combustible gases can be condensed into a combustible liquid, called pyrolysis oil (bio-oil), though there are some permanent gases (CO2, CO, H2, light hydrocarbons) some of which can be combusted to provide the heat for the process. Thus, pyrolysis of biomass produces three products: one liquid (bio-oil), one solid (biochar) and one gaseous (syngas) (see more ).
Biochar is a solid and charcoal-like substance. Biochar contains 70% carbon. It is used as soil enhancer to increase fertility, prevent soil degradation and to sequester carbon in the soil. Furthermore, most of the technologies that make it work are already quite advanced. But at present, the technical system of biochar production by biomass carbonization is still not perfect, and further in-depth research is still needed. There are other disadvantages. For a start biochar sometimes disturbs the physical and chemical balances of nutrients in the rhizosphere. The rhizosphere is the zone of soil surrounding a plant root where the biology and chemistry of the soil are influenced by the root. Moreover, biochar generally helps the growth of undesirable weed and biochar production is relatively expensive (see more ). In addition, the biomass should not come from feedstock because otherwise there is a competition with food (see more ).
Conclusion
There are several carbon sequestration technologies. Each technology has its pros and cons. Overall, most of them are not ready to be implemented on a large scale and if they are, they are still relatively expensive. The most attractive options in terms of costs are reforestation and afforestation. In our next report, we will see if the other geoengineering options are more promising.